Research
Dr. Harb's research group studies, develops, and optimizes electrochemical systems. Systems of interest include energy storage and conversion devices, micro- and nanofabricated electrochemical devices, and the fundamental processes that govern electrodeposition and dissolution. This page briefly describes research projects that are currently underway in our laboratory.
Coating Technology for Next Generation Corrosion Protection
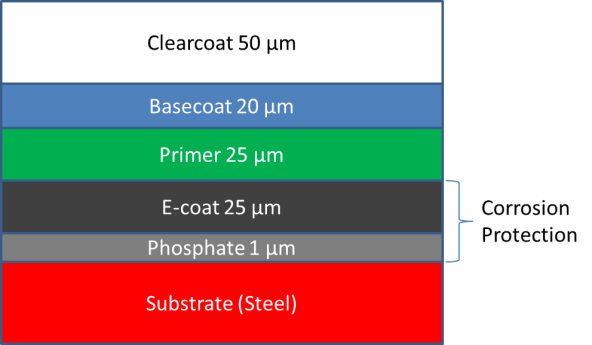
Current practice in the automobile industry is to electrophoretically apply a paint layer (E-coat) to the car body after welding to provide corrosion protection and a base layer for subsequent paint layers. The E-coating process is performed in large baths on the assembly line at potentials of 200V or more (DC). For complex shapes, the coating first forms where the current density is the highest; it then moves to the more difficult areas as the coating builds up and slows down the local rate where the film has already formed. A key challenge is the application of layer of sufficient thickness in recessed areas. There is also opportunity to optimize the process to, for example, reduce time in the bath or increase energy efficiency. Much of the process design to date has been done empirically. Our work seeks to address these important issues by developing an increased understanding of the fundamental processes that govern the E-coat process, and incorporating that understanding into next generation mathematical models.
Funding: CD-adapco
Graduate Student: Fardin Padash
Use of DNA Origami for Bottom-up Fabrication of Molecularly Templated Nanocircuits
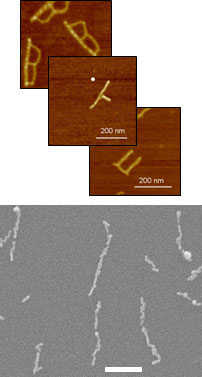
DNA-based self-assembly is becoming increasingly attractive for the fabrication of complex architectures with nanometer precision. This approach takes advantage of the ability of biopolymers such as nucleic acids to spontaneously self-assemble into structures, and has seen remarkable advancement with fabrication of a variety of 2- and 3-D nanostructures. DNA origami is the technique that we use for designed folding of DNA into desired shapes with known attachment sequences. DNA is especially appealing because of its high aspect ratio, narrow linewidth (~ 2nm) and ability to form complex nanostructures through base pairing that allows the designer control at the molecular level. Our work uses DNA-based structures as molecularly addressable templates upon which the metal and semiconducting materials needed for electronic devices can be formed. Electrochemical processes are used to create and modify the required materials on the DNA templates. Consequently, DNA-based fabrication provides an alternative to current microelectronic fabrication techniques that has the potential to reduce feature sizes and form complex structures that are beyond the limits of the prevailing fabrication methods.
Collaborators: Adam T. Woolley, Chemistry, BYU; Robert C. Davis, Physics, BYU
Funding: Semiconductor Research Corporation, National Science Foundation (completed NIRT project)
Graduate Students: Bibek Uprety, Michael Stoddard
Link to previous NSF-funded ASCENT project
Galvanic Corrosion of Magnesium
Magnesium
is a lightweight metal with good structural properties that has the
potential for increased use in automobiles. There is also interest
in the use of Mg for batteries and in hydrogen generation systems. A
key obstacle to the use of Mg is its corrosion susceptibility. While
several previous studies have sought to understand the mechanisms that
control the dissolution rate and surface morphology of Mg during
corrosion, much less is known about the galvanic corrosion of magnesium.
The purpose of this project is to investigate the impact of galvanic
coupling on Mg corrosion, and the extent to which such coupling
influences both the morphology and rate of corrosion. To do this,
we combine carefully designed experiments with mathematical simulations
to increase our understanding and to enable quantitative prediction of
the corrosion behavior.
Funding: CD-adapco
Graduate Student: Dila Banjade
Nanostructured Battery Electrodes for Next Generation Energy Storage
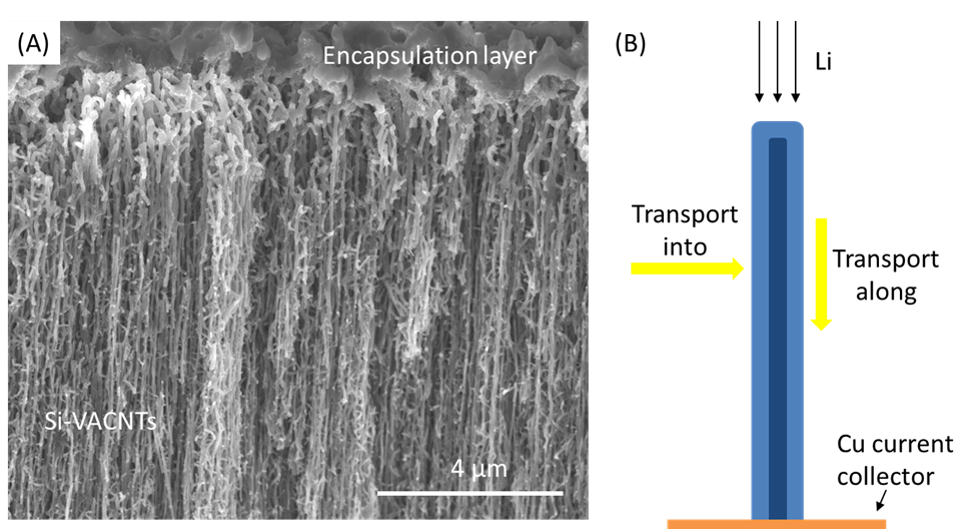
Si-based anodes are being explored for use in advanced lithium ion batteries due to their capacity and energy density, which exceeds that of current graphite anodes. However, there are several important factors that influence and often limit the cycling performance of these electrodes, including large silicon volume changes and extensive SEI formation. Nanostructured electrodes have been shown to effectively reduce the negative impact of volume expansion; however, the use of nanostructures can greatly increase the electrode/electrolyte surface area and lead to greater SEI formation. Consequently, several strategies have been developed to reduce the area available for SEI formation while maintaining the benefits of a nanostructured material. In this study, we use silicon-coated vertically aligned carbon nanotube (Si-VACNT) electrodes to examine the role of Li transport in a system where encapsulation has been used to reduce the surface area of the nanostructured electrode that is exposed to the electrolyte. This system is ideal for studying the impact of an electrolyte-blocking layer due to its well-defined geometry and high aspect ratio.
Collaborators: Robert C. Davis and Richard R. Vanfleet, Physics, BYU
Funding: National Science Foundation
Graduate Student: Juichin (Rita) Fan
Viologen-catalyzed Electrochemical Conversion of Biomass for Sustainable Energy and Products
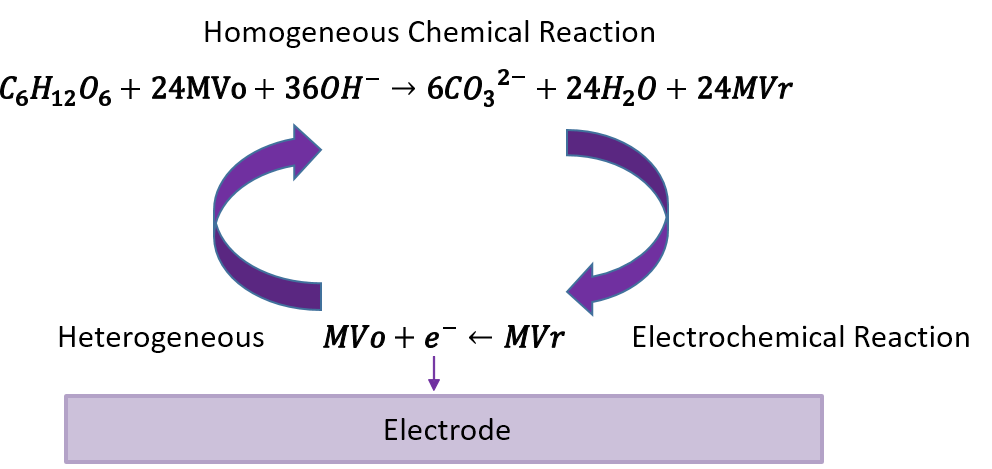
This work seeks to use a homogeneous viologen catalyst to convert glucose to carbonate in a biofuel cell. This viologen-based catalyst functions as a mediator or “electron shuttle,” and initial experiments show that it has the potential to enable almost complete conversion of the carbohydrate, depending on the experimental conditions. Calculations indicate that, with use of this catalyst, it should be possible to achieve conversion rates that are much higher than those reported for traditional biofuel cells. Also, because the proposed carbohydrate fuel cell operates at high pH, the end product of the carbohydrate oxidation is carbonate rather than carbon dioxide. It should be possible to remove the carbonate from the system as a product. Therefore, the system under study may actually result in a net decrease of carbon dioxide in the atmosphere, since biomass consumes carbon dioxide during growth and the carbon from the biomass exits the fuel cell sequestered as carbonate rather than escaping as CO2. The goal of this EAGER project is to demonstrate proof-of-concept regarding the potential for near-complete conversion of glucose at high rates.
Collaborators: Randy S. Lewis, Chemical Eng., BYU; Gerald D. Watt, Chemistry, BYU.
Funding: National Science Foundation
Graduate Student: Meisam Bahari